Bacterial adhesion
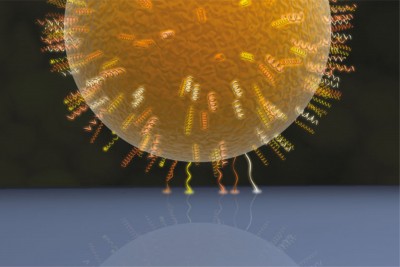
These are a few of the many questions we are trying to answer in our group. To answer them, we mainly apply atomic force microscopy (AFM)-based force spectroscopy, using either bacterial clusters or single bacterial cells as force sensors. In both cases, cells remain viable during the measurements which can be confirmed by live/dead staining.
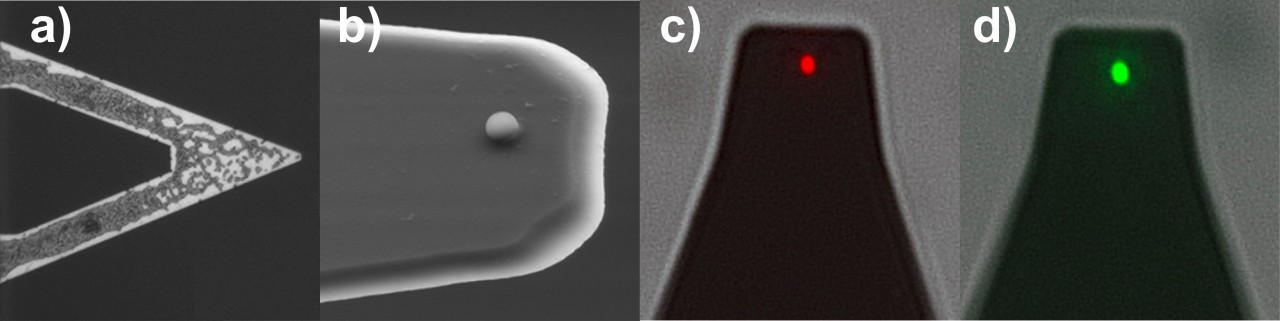
(the red shining cell was killed, while the green cell is viable).
In our experiments, we typically record force-distance curves providing us quantitative distance-dependent values of the interaction between the bacterial cells and the surface. For example, we can extract the adhesion force, adhesion energy, and the rupture length from retraction parts of the force-distance curves. In the approach part of the curves, for hydrophobic surfaces, we also see a snap-in event that can be characterized in terms of the maximal occurring force and the distance at which it starts. Besides force spectroscopy with bacterial probes, we also perform flow chamber measurements, with the advantage that many more single cells can be measured simultaneously.
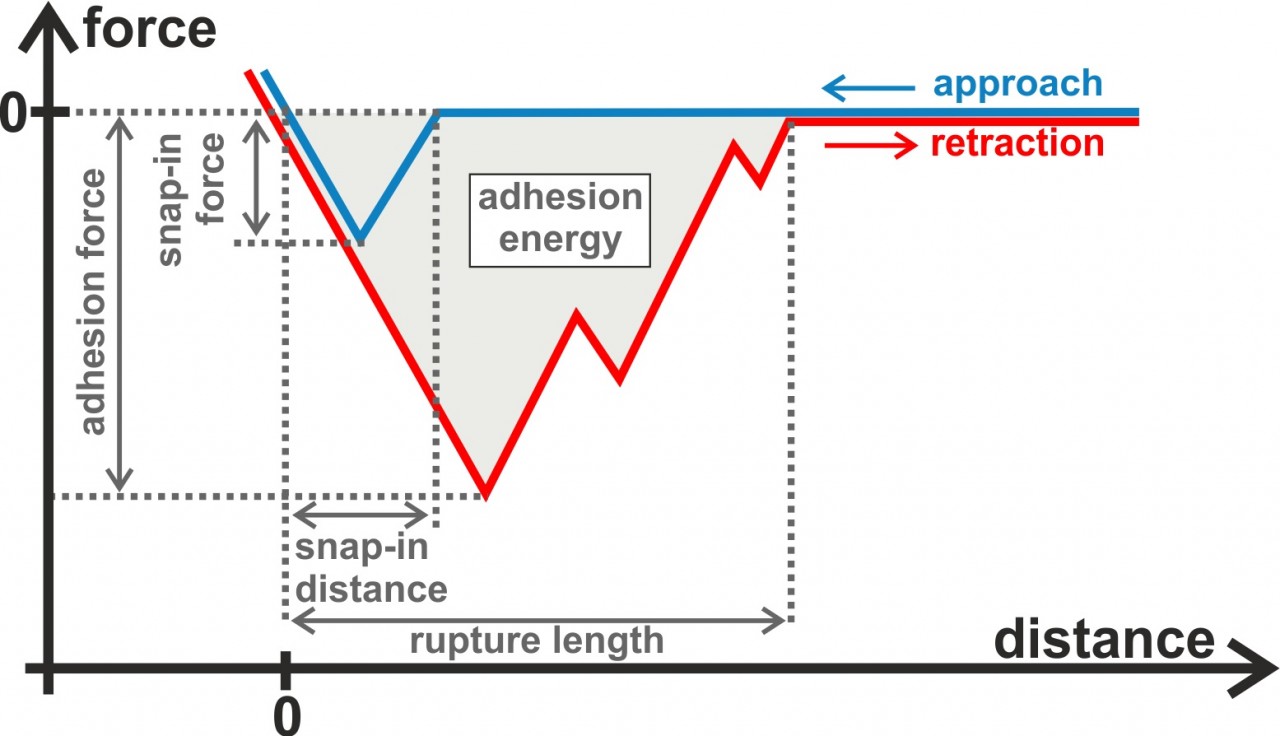
Our experiments led to a new model of bacterial adhesion that almost solely relies on the tethering of cell wall macromolecules. When approaching the surface, macromolecules bind to the substrate consecutively, and, if there are enough of them, they pull the whole cell body to the surface. Upon retraction, the macromolecules lose contact with the surface one after another, leading to the typical shape of bacterial force-distance curves that depend on the number of tethered macromolecues. Using this model, experimental results could be quantitatively reproduced by Monte Carlo simulations.
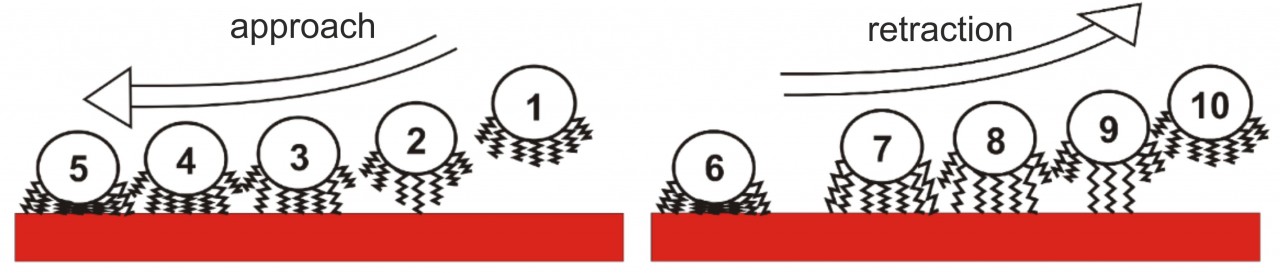
Our experiments also showed that for the adhesive strength of staphylococcal cells, such as S. aureus, S. carnosus, both short-ranged surface forces and long-ranged van der Waals-forces play a role. For example, we demonstrated that the cells adhere much better on hydrophobic surfaces than on hydrophilic surfaces. Using this knowledge, we were able to determine the contact area between staphylococcal cells and flat surfaces. Surprisingly, the size of this contact area is not correlated to the adhesive strength of the respective cell.
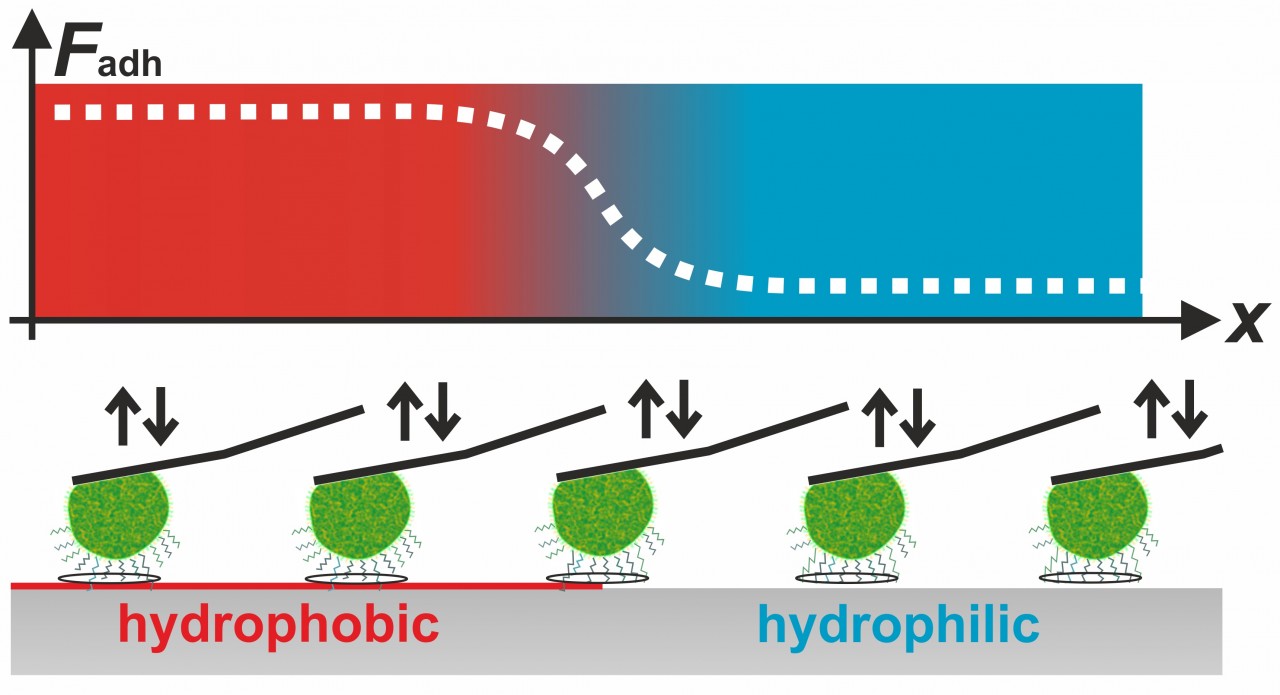
We also perform experiments with streptococcal cells with focus on their adhesion to close-to-nature surfaces, such as hydroxyapatite (HAP), the mineral component of teeth. We showed that S. mutans cells are well adapted to their natural oral habitat because their ability to adhere to HAP increases when exposed to saliva. In another study, we proved that the adhesion of oral bacteria to HAP is reduced when the surface was fluorinated before as it is achieved by many oral care products.