Graphen und Boronitren (White Graphene)
Graphene represents a single atomic layer of sp2-bonded carbon atoms which excels by its unique electronic properties. The band structure of this two-dimensional honeycomb-lattice provides a special features close to the Fermi energy, namely a linear band dispersion. In the vicinity of the so-called Dirac point, the conical shape of the band structure causes the electrons to be relativistic particles of nearly vanishing mass, resulting in ballistic transport close to the µm-range. Graphene is therefore believed to sound the bell for replacing silicon when crossing the border to future nano electronic devices. However, graphene's mystique of being a magic material is also related to its mechanical properties, such as its extraordinary tensile strength, making it stronger than the strongest materials known so far, such as, e.g., Kevlar or any high performance steel. Therefore, future applications of graphene also aim at its use as solid lubricants in tribology or as raw material in composites. Today, the long list of potential application of graphene is hard to survey: In bionics, graphene may act as a sensor for different biopolymers. In energy storage, graphene may act as electrodes in electro chemical super capacitors. In surface science, graphene may act as a coating with full or partial wetting transparency. In environmental science, graphene may act as a highly efficient nano filter. In relativistic quantum mechanics, graphene may provide a full, but low-cost QED lab, etc.
Irrespective of the particular application, large scale synthesis of graphene is required when using this material in future everyday life. Geim's and Novoselov's original synthesis route as based on the exfoliation of HOPG (Highly Oriented Pyrolytic Graphite ) provides high quality graphene of only several µm2, a scale that is sufficient to do basic research in the lab but far from being sufficient for mass production on a industrial scale. For example, the labor costs for a PhD student producing 1 cm2 of graphene via exfoliation can easily exceed 106 €. Therefore, nature is asked to do graphene synthesis by its own via the mechanisms of self-assembly.
One method to provide large-scale graphene is based on the epitaxial growth on transition metal surfaces via the chemical vapor depositions or the liquid precursor deposition of carbon containing precursors, as applied in our group.
Boronitrene (also called "White graphene" or h-BN monolayers) is an isostructural and isoelectronic analogue of graphene with the honeycomb lattice built by to different sublattices - one made by N atoms and one made by B atoms. Its mechanical properties are quite similar to that of graphene but with respect to its electronic properties there is a distinct difference. Since the boronitrene lattice is built by two different sublattices, a band gap of about 5.5 eV opens. Therefore, boronitrene represents a one-monolayer insulator that is predicted to replace silicon oxide in future nano transistors. In combination with graphene, Intel's former slogan may read as follows:
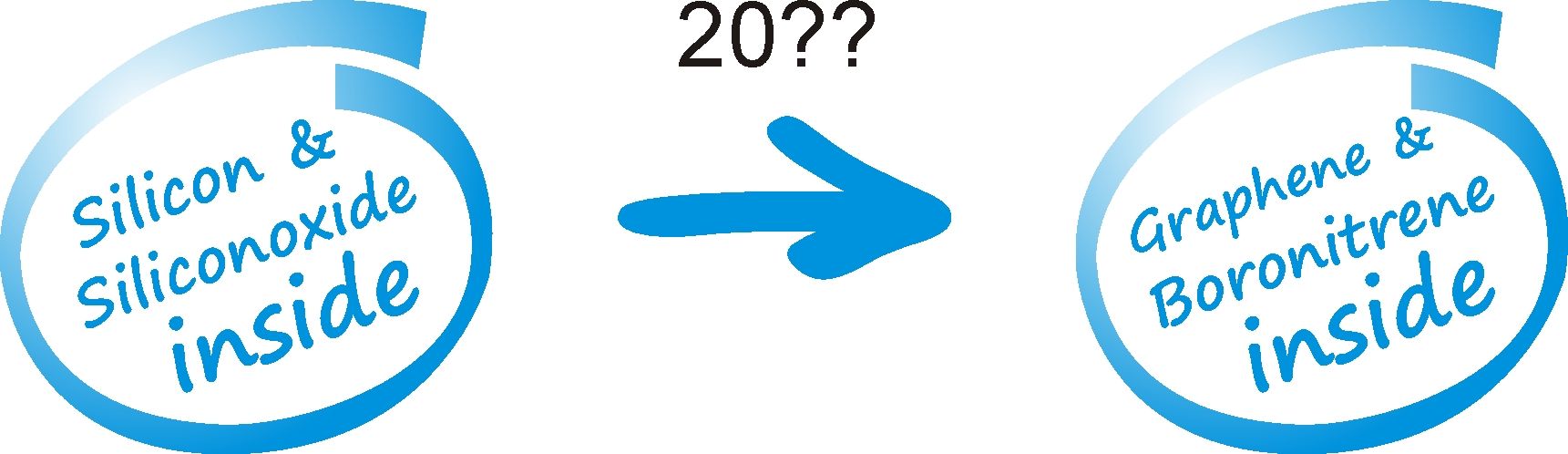
Fig. 1: This vision of the future is expected to be just a matter of time ...
 Icon.jpg)
Fig. 2: a) Band structure of graphene, according to the simple Tight-Binding -Ansatz by Wallace (P.R. Wallace, Phys. Rev. 71 (1947) 622) with conduction and valence band attaching at the Fermi energy at the K points of the Brillouin zone (Dirac points). b) Part of the 3d-Fermi surface of clean Ir(111), as probed by Fermi Surface Mapping (FSM, He-I excitation). c) FSM after the formation of one monolayer graphene on Ir(111) with the Dirac points clearly visible at k‖ = 4π/3agraphene = 1.703 Å-1 (Figure according to F. Müller et al., Phys. Rev. B 84 (2011) 075472).
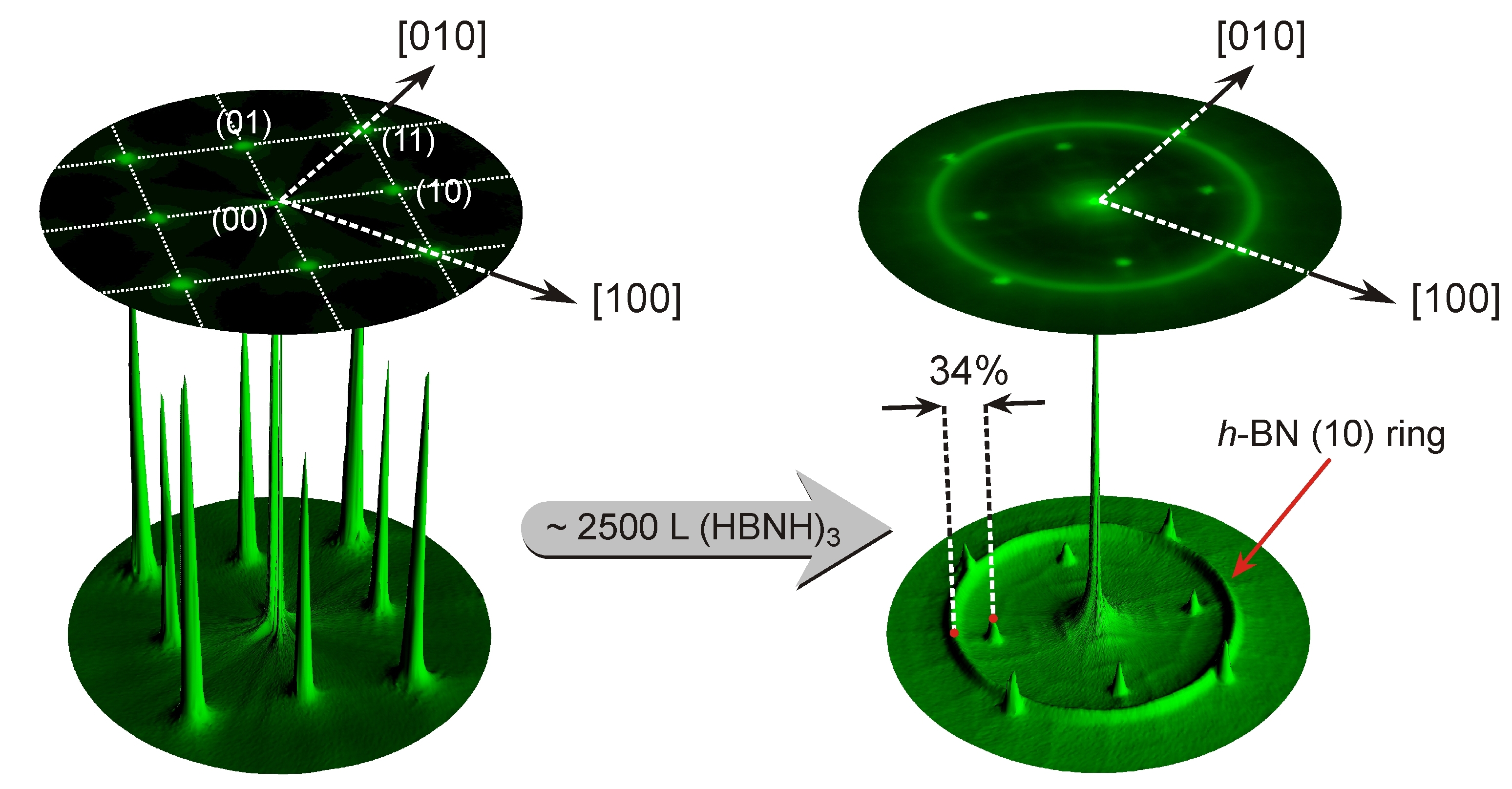
Fig. 3: LEED (Low-Energy Electron Diffraction) pattern of a clean Ag(001) surface (left) and after formation of one monolayer of boronitrene after CVD of borazine at 850 K (middle). The metrics in the polar intensity plot (right) give clear evidence that the ring like structure result from boronitrene domains of arbitrary azimuthal orientation (Figure according to F. Müller, S. Grandthyll, Surf. Sci. 617 (2013) 207).